Within Part One of this series we learned that fringing
reefs are those reefs that develop in shallow waters along the coast of
tropical islands or continents. Fringing reefs are generally narrow
platforms a short distance from shore and do not contain substantial
lagoons. They can however contain expansive areas of nearshore shallow,
sandy substrates receiving intensive sunlight with little to no other
structures that could shade such areas. Such a landscape provides the
conditions that allow for the formation of tropical seagrass beds
provided that a suitable and somewhat stable sediment is present.
This article will examine a natural seagrass ecosystem as found just
offshore of Mactan Island, The Philippines. I will discuss its
diversity of plant species, its inhabitants, both temporary and
permanent residents, and its productivity. During my research for
this article it became apparent that I would also have to include
information on the deep sandbeds that are vital to these habitats. As
such, there will also be a short review concerning the nutrient
dynamics and the sand infauna of such sediments.
Seagrass - The primary producers
With four families, twelve genera and about sixty species (
Sullivan 1994)
the seagrasses
have been able to colonize all relatively warm locations providing a
unique and very diverse habitat regardless of the species or mixture of
species found. Although there are many seagrass beds comprised of
single species found elsewhere in the world, here in the
Philippines there is high diversity of species (seven to nineteen
species according to multiple sources) and the grassbeds are most
always of mixed species.
Locally the most common species are the very large bladed slow growing and long lived (10 years)
Enhalus acoroides,
the short wide bladed
Thalassia
hemprichii, the short very thin bladed
Syringodium isoetifolium
and the short, paddle shaped
Halophila
ovalis.
Each of the four species plays a role in the formation of the
grassbed's climax canopy. With nearby open sandbeds, the
Halophila acts
as the pioneering species, being the first to establish itself in
uncolonized sand acting to anchor the sand and preparing it for the
Thalassia and
Syringodium
species to follow through rhizome growth. It is only when sufficient
growth by the previous species has stabilized and enriched the sandbed
through their leaf litter that the large
Enhalus
species
establishes itself, which it appears to do more frequently through seed
dispersal than by rhizome growth. I have only observed this large
species being located in the central regions of the grassbeds
indicating to me that it or its seeds were late arrivals onto the scene,
giving the shorter lived, faster growing species time to prepare for
its arrival while having spread far beyond their point of origin.
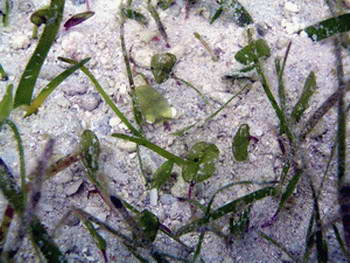
The paddle shaped
Halophila
ovalis having pioneered open sand substrate allowing
Thalassia hemprichii to
follow.
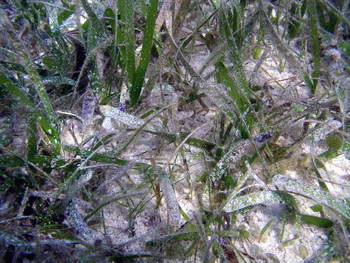
A young seagrass bed having been fully colonized by
Thalassia hemprichii
and
Syringodium
isoetifolium thus overgrowing and pushing out the pioneer
Halophila ovalis.
The thick layer of leaf litter has yet to accumulate as found
in mature beds.
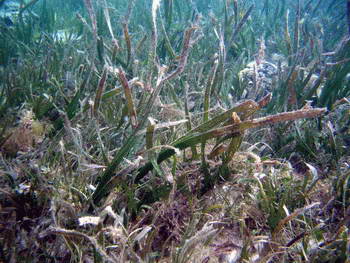
A mature seagrass bed containing multiple species of seagrass and
having developed a thick layer of leaf litter. The fully developed
canopy also provides yet another habitat utilized by many fish and
invertebrate species, some being full time residents while others
follow the tide in from the deeper reef to hunt for food within these
very rich hunting grounds. What seems most important for the
associated species is the provision of shelter and food supply
resulting from their extraordinarily high rate of primary production.
The formation of
coastal seagrass beds
also help to provide the required conditions for the fringing coral
reefs by slowing the flow of water and allowing sedimentation to occur before such
particulates can become a hazard to the corals. Seagrasses also provide
coastal zones with a number of other benefits including wave
protection, oxygen production and protection against coastal erosion by
anchoring the sediments in place and preventing their drift. The
nursery habitat that is created and sustained by the seagrasses is an
important contribution to the fisheries, greatly adding to the number
of fish that reach adult size having been afforded the
protection
and food provided by the seagrass ecosystem.
Seagrasses are monocotyledonous vascular flowering plants. They
are unique in that they are submerged in the seawater, possess a
rhizome/root system with stems buried in a soft substrate, have
vegetative and sexual reproduction and have flowers fertilized by
water-borne pollen. Seagrasses are the only true marine plants as
all other "vegetation" found in the ocean are algae. While not a true
grass, they are called grasses simply because their long, green leaves
superficially look like the terrestrial grasses from which they evolved
from.
Anatomy
of a seagrass (
Syringodium isoetifolium)
Seagrass sediments - A place of complex nutrient dynamics and home to many species.
The seagrasses are considered to be ecosystem engineers since they
partially create their own environment simply by growing and living in
most soft, sandy sediments. Once established by the pioneer species,
their extensive root systems take up nutrients which are then
transported to the leaves.Upon the leaves’ death and detachment,
they will settle on the surface of the sediment and through
decomposition not only return a portion of its sequestered nutrients to
the sediment, but will also create the acidic conditions through
decomposition that releases even more adsorbed nutrients from the
sediment in which it grows. All of the nutrient exchange is also
utilized by the multitudes of infauna that through their own actions
within the sediment contribute to the distribution of nutrients and
thus the growth of the seagrasses.
I
feel it is noteworthy to point out that other studies done in locations
outside of the Indo-Pacific region have come to different conclusions
concerning the nutrient dynamics of seagrass sediments. This may be due
to differing sediment compositions as well as the different seagrass
genera found in those locations. Not all seagrasses have the same
requirements nor the same abilities in nutrient
extraction/transportation. Since this article is examining a
Philippine (Indo-Pacific) seagrass habitat, I have tried to use only
the reference material that pertains to these locations. This
should not pose a problem for the aquarium hobby as the majority of our
aquarium systems are based on the Indo-Pacific regions and
their calcium carbonate sediments.
I also want to stress
the fact that the nutrient dynamics involved in any seagrass ecosystem
is extremely complex and not something I can or am willing to fully
explore in a single hobby article. I will however do my best to
touch upon the most obvious of the actions involved as they do pertain
to our keeping of marine aquaria.
As with any plant,
light and nutrients are the primary requirements for growth. With the
surrounding sea water often having undetectable amounts of dissolved
nutrients, the seagrasses derive the majority of their nutrients
directly from the substrate by way of their root. Although the
leaves can also uptake nutrients from the water their primary purpose
appears to be conducting photosynthesis and storing nutrients
transported by the roots. The nutrient concentrations within the water
are usually so low that uptake by the leaves is considered
insignificant relative to root uptake of nutrients from the sediment
(Erftemeijer 1993). As with any plant or algae that utilizes both
phosphorous and nitrogen, they can be limited by not enough of one or
the other. How much of one or the other is available is determined by
numerous factors, most of which involve the geochemistry of the
sediments that the seagrass finds itself growing along with the
availability of organic matter that is broken down through
decomposition, the primary source of both nitrogen and phosphorus
regardless of the sediment's composition.
Any good farmer
knows that phosphorous and nitrogen within the soil is the key to a
good crop in nutrient poor soils, hence the heavy use of fertilizers in
farming operations. This holds true for seagrass as well.
The ability of a substrate to provide the essential dissolved nutrients
has been shown to be determined by the composition of the sediment
(Short 1987) in of its composition, either terrigenous (land-based
eroded rock) or calcium carbonate. The grain sizes also determine the
nutrient dynamics involved. It has been shown (Erftemeijer 1993)
that Indo-Pacific, near-shore sediments comprised of terrigenous
material has a significantly higher pore water concentration of
nitrogen compounds than the calcium carbonate-based sediments while the
reverse is true of phosphorous compounds. This can be explained
by the geochemistry found to occur within the various sediments
and at varying depths within those sediments.
Data as given by Erftemeijer P.L. (1993),
Sediment-Nutrient interactions in tropical seagrass beds
The two sedimentary environments investigated by Erftemeijer showed
considerable differences in sediment composition and nutrient
availability. Total P and N were much higher in the terrigenous
sediment in comparison to the nearly 100% calcium carbonate sediment
The difference was attributed to the terrigenous study area
being near a river inlet causing an increase of organic matter from
terrestrial sources. However, the exchangeable phosphate was considerably higher in the
calcium carbonate sediment and was attributed to the much stronger
adsorption affinity of the carbonate matrix to phosphate in comparison
to the terrigenous sediment.
Additionally, the apparently
high levels of phosphate within the upper few centimeters of the
carbonate sediment can be attributed to the carbon dioxide and acids
produced as a result of aerobic decomposition of organic material and
oxidation of reduced sulfur compounds. These acids may cause the
dissolution of calcium carbonate and the phosphate that had been
adsorbed onto the calcium carbonate, resulting in a net enrichment of
porewater phosphate.
Within the upper few centimeters of calcium
carbonate sediments, bacterial fixation of
N2 (
Capone
1992) accounts for a large fraction of the NH4 produced within or
released from the upper layers of the sediments, having a turn over
rate of less than twenty four hours.
Capone has found
that denitrification can be detected, even in apparently oxygen
rich sediment, possibly accounting for the lowered nitrogen
content in relation to phosphate content and thus limiting seagrass
growth to being more dependant upon phosphate when growing in calcium
carbonate sediment. Again, the reverse is true when the sediment is
comprised of terrigenous materials.
The relatively high availability of phosphate in porewaters from
coarse-grained carbonate sediments in seagrass beds found within the
study (
Erftemeijer 1993)
is in contrast to the general assumption that seagrass growth on
carbonate sediments is phosphorus limited (
Short 1987). But that study
was working in fine-grained sedimentary environments (carbonate mud and
silt) while another study (
McGlathery 1992) found evidence of
nitrogen limitation. Given the apparent discrepancies between nitrogen
and phosphate limitations on seagrasses within the various studies done
to date, Erftemeijer concludes that the grain size of the sediment is
one of the primary factors determining the availability of phosphorus
in a tropical carbonate sediment. This is something to keep in mind when
constructing a live deep sand bed for an aquarium.
Life within the sediment - The Recyclers
A few members of the sandbed infauna :
Foraminiferans
and their remains are clearly the most abundant of the visible life
forms found within the sediment. Not surprising given that Dr. Ron
Shimek has sampled foraminiferans with a density of over 70,000 per
square yard of ocean bottom.
Polychaete worms
and nematodes are also found in great abundance. Most are microscopic
containing both predator and prey species. By just their sheer numbers
and relative mobility, they account for a great deal of the nutrient
processing and recycling within the sediment and by their movements through the sediment help to turnover
the sediment's layers.
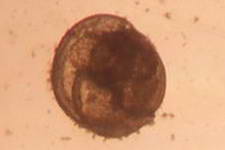
A barnacle cyprid
Microscopic Gastropod
A Gastropod
veliger
Two of the largest sediment dwellers that ingest sediment grains
digesting any organics attached to the grains and any detritus that may
have been pulled into the sediment. Their movement also irrigates
and disturbs the sediment.
Holothuridea sp.
Synaptid
sp.
Epiphytic Organisms - Important producers within seagrass habitats. 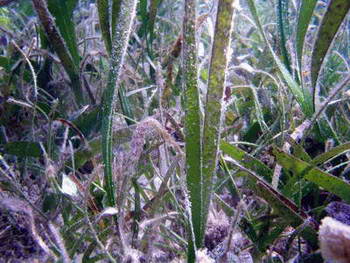
The high productivity of seagrass beds is the product of not only the
seagrasses but also a variety of epiphytic organisms that use the vast
amount of surface area provided by the seagrass leaves on which to
grow. The most abundant of the epiphytic organisms are the
microalgae, providing as much as 46% of the autotrophic production of
seagrass beds. Since seagrasses are not known to produce any toxins or
have any mechanisms to control the attachment and growth of
epiphytes, epiphytes can be found on all exposed parts of the
seagrass.
Though the presence of epiphytes on the leaves of seagrasses is a
natural phenomenon and contributes to the productivity of a seagrass
ecosystem, eutrophication can cause abnormally high rates of epiphytic
macroalgae and microalgae growth leading to the complete shading of the
seagrasses and their subsequent loss.
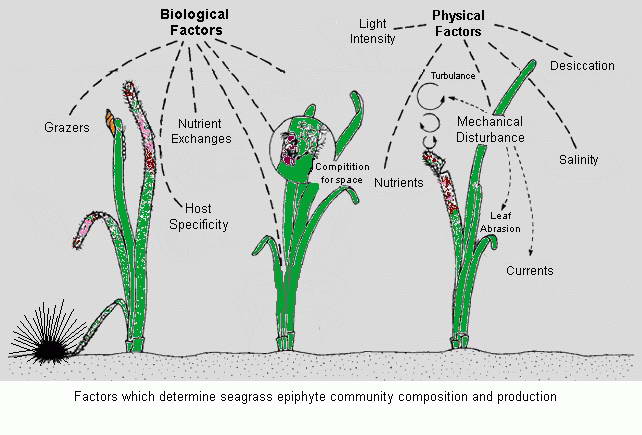
Algae are not the only organisms that quickly take advantage of
any substrate that affords them a position in the sunlight or water
currents much to the detriment of the smothered individual leaf.
The sacrifice of individual leaves creates a much greater benefit
to
the seagrass meadow as a whole and is responsible in large part
for the high rate of productivity which in turn fuels the complex
ecosystem and nutrient food web that extends far beyond the
confines of the seagrass meadows.
Epiphytic growth
while seemingly detrimental to the seagrass may also benefit those
plants that grow in areas that tidal movement exposes them to the air.
With a coating of epiphytic life forms, moisture retention is
enhanced and may allow the seagrass to avoid desiccation (
Bell 1997).
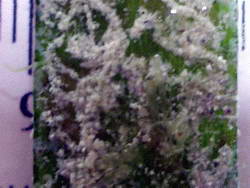
As each leaf is covered in epiphytes, the ability of the leaf to
perform photosynthesis is reduced and reaches a point where the leaf is
of no use to the plant anymore. The leaf is cast off, along with
any epiphytes unlucky enough to have settled on what seemed a
permanent home. The cast off leaf now further enriched with other
life becomes part of the leaf litter mat and is acted upon by bacterial
and fungi creating the detrital matter that so many other organisms
find of use. Having lost a leaf, the plant then pulls even more
nutrients out of the sediment to create a new leaf to regain its
photosynthesis capacity and makes sediment bound nutrients available
once again. In turn, yet another new surface area arises for the
epiphytes to colonize, and so the circle begins again. With
individual leaf life spans having been estimated to be anywhere from 3
to 10 days, there is a vast amount of organic material that a seagrass
meadow is producing in a single week.
Benthic microalgae
(microphytobenthos) while very important in other shallow ecosystems do
not contribute to the biomass and productivity in any significant
amount within a mature seagrass bed. The lack of benthic microalgal
activity is attributed to the sediment being shaded by the seagrass
leaves, its leaf litter and the thick layer of detritus that blocks the
sunlight and prevents photosynthesis from occurring. In a
developing seagrass bed the benthic microalgae would play a larger role
in nitrogen fixation within the sediment since it is unlikely that a
sun blocking layer of leaf litter and detritus would accumulate for
quite some time. This microalgal layer may account for the added
nutrient enrichment that the pioneering seagrass species need to gain
new territory.
Epiphytic & Off Shore Drift MacroAlgae - Damaging intruders or contributors?
A tropical seagrass meadow will also likely contain macroalgae species (Bell 1997)
that have either grown as epiphytes on any of the available surfaces or
having been carried into the area by water currents and snagged on the
seagrass blades. In mature seagrass meadows, the unstable leaf
litter does not present many substrates on which to attach other than
the seagrass leaves or the larger exposed rock fragments.
I
have noted marked seasonal variations in the abundance of macroalgae
within the seagrass meadows. During the monsoon season there is
an obvious increase in the amount of macroalgae present due
to frequent storms that create enough force that detach epiphytic
or benthic macroalgae and drive them into the seagrass
areas. During the relatively dry season, storms are rare allowing
the epiphytic macroalgae to remain where they have attached or
settled.
The storm driven macroalgae that finds
itself stranded within the seagrass meadows at the end of the monsoon
season is most often left undisturbed during the dry season allowing
the algae to stabilize and grow only to be torn away at the start of
the next monsoon season. The macroalgae, having grown larger, now
presents more surface area to the water currents. I believe this
and the lack of a stable substrate ensures that the macroalgae do not
dominate or destroy the seagrasses and the result is that they are
mostly transitory.
During the relatively brief stay within
the seagrass meadow, the macroalgae will continue to remove nutrients
as they would anywhere else that they can grow. They take from the
local nutrient pool only to transport the nutrients elsewhere when the
season changes and the macroalgae is set adrift once again. Eventually
the macroalgae's luck will run out and they will be washed up
onshore, snagged on the coral reef eaten by herbivores or sink into the
abyss. Either way the macroalgae has transported a fraction of the
seagrasses productivity elsewhere. Being seasonal and dependant
upon the severity of the monsoonal storms, how much nutrient
transportation takes place can be highly variable from year to
year.
I have not observed any detrimental affects of
any significance by the epiphytic or drift macroalgae as they are
transitory in nature. Any damage done is restricted to small
localized areas and is temporary (i.e. the macroalgae can shade/smother
an individual seagrass plant and cause its demise). If the macroalgae
is an epiphyte upon the seagrass leaves, the loss of the seagrass can
also mean the loss of the macroalgae as it is dropped into the leaf
litter. If the macroalgae is adrift, the loss of the seagrass
leaves will most likely allow the macroalgae to drop down onto the leaf
litter and find itself becoming shaded and smothered, as well as
possibly being consumed by the local herbivores.
This all
points to transient macroalgae having their nutrients either
transported into the seagrass ecosystem by drift, or having their
nutrients and any additionally gained nutrients through growth
being transported out of the seagrass ecosystem or simply being
recycled back into the seagrass ecosystem through herbivorous action
and decay.
Near-Shore Ulva spp. - Now you see it, now you don't.
Ulva spp. are another drift macroalgae that can also affect the seagrass
community. Where the drift macroalgae mentioned previously
originate from further offshore in relation to the seagrasses locale,
the Ulva sp. originate near the shoreline prior to the seagrass meadow.
Following the seasonal cycle of the tropics, of which there are
only two, a wet monsoon season and a dry season both of equal duration,
limits the impact that these algae may have on the seagrass to a few
months of the year when heavy rains wash the land and create eutrophic
conditions near shore.
I have often wondered at how such a loosely attached and often free floating algae
could seem to completely disappear for many months only to make a rapid reappearance
seemingly out of no where, hence the title of this section. The
answer lies within its life cycle and within the local seasonal variations.
Ulva follows a reproductive pattern called alternation of generations, in which it
takes two generations to complete its life cycle, one that reproduces sexually and one that reproduces
asexually. Although mature members of both
generations look the same to the naked eye, microscopic chromosomal differences
distinguish one from the other. The first generation, which has
two complete sets of chromosomes (
2n), the second generation has only one set of chromosomes
(n). The first generation, called
the sporophyte, undergoes asexual reproduction to form spores, tiny reproductive
cells that develop into mature individuals called gametophytes. Gametophytes
produce gametes, male and female reproductive cells that fuse together during
fertilization to produce a zygote, an organism with two complete sets of
chromosomes that matures into a sporophyte, thus completing the life cycle.
Ulva spp.are
relatively simple when compared to more advanced algae and vascular
plants. They do not differentiate into tissue layers or show much
specialization among cells, making them a colony of like cells
forming new cells perpendicular to the surface which gives this species
their distinctive thin "tissue paper" appearance. Since each
cell, including the reproductive cells, are capable of photosynthesis,
the uptake of nutrients and adjustments to light intensity is very
rapid.
Due to their high nitrogen requirements and their limited ability to store nitrogen,
Ulva
distribution is nitrogen limited and explains their seemingly
quick appearance and subsequent disappearance after heavy rainfalls.
Such occurrences make
Ulva spp.
good bioindicators of local water quality pertaining to its level of
nitrogen enrichment. In such areas of constant enrichment,
Ulva spp. become a permanent feature and can easily smother all benthic organisms found below it.
It is worth noting that there are no local
Ulva populations
here that could account for the sudden growth observed during periods of
eutrophication after heavy rainfalls. This leads me to conclude that
their zygotes are being carried in from distant locations that do contain
permanent populations due to year round nitrogen
enrichment as found near sewage discharges or river inlets.
Once
nutrient conditions favor local growth, these algae can
make a very sudden
appearance near the shoreline. As they grow they will begin to settle on top of the bare sand and rubble substrate
nearshore to the seagrass meadow and remains near shore. I at first attributed the shallow water propensity of
Ulva
to one of light intensity but have since learned that its appearance in
near shore shallow water is simply a matter of available nitrogen
through terrestrial runoff and only remains near shore due to a lack of
sufficient wave disturbance. A
study of
U. lactuca (
Hansen 1992)
has shown that this macroalgae can compensate for reduced light
intensity by its ability to rapidly increase its cellular chlorophyll
content and has its highest rate of growth with a mere 10% of available
ambient light. These facts do not bode well for the marine
habitats as the only limiting factor of
U. lactuca
appears to be the availability of nitrogen, making any relatively
shallow habitat vulnerable to being smothered by its rapid growth
should nitrogen eutrophication occur over extended periods of time.
With their appearance and having reached a
sufficient size to present enough surface area to be uplifted by storm
driven waves the algae is then carried offshore by tidal action where they
become
entangled with the seagrass leaves. I have not noted any
detrimental affects to the seagrasses other than the localized loss of
single leaves due to shading by these macroalgae.
Upon the loss of the
leaves, the attached
Ulva
are either dropped down into the leaf litter
where local grazers consume it or if left uneaten, will in a matter of
days die as any available dissolved nitrogen is quickly consumed.
Upon decomposition its limited nitrogen content does not
appear to
have any long term consequences as
I have observed this local seasonal phenomenon since 2004 with no
apparent
loss of seagrass cover to date.
Bacteria / Fungus - The workhorses of all environments.
Other than the much larger fish and animal grazers, most other animals
can not directly consume seagrass due to its fibrous composition. The
bacteria and fungi are the dominant consumers of seagrass primary
production once such production has been added to the leaf litter and
begins decomposition. By their actions upon the cast off leaves
they break down the fibrous material making it available to the
majority of animals that otherwise would not be able to utilize
seagrass production. Bacteria not only use organic matter
supplied by the seagrasses, but also any organics that have been
recycled from animals and previous bacterial activities.
While the bacteria and fungus first make the cast off seagrass blades
available to most other animals through decomposition, they also
process the waste from the animals that benefited from their originally
breaking down the seagrass production. They also utilize the
byproducts of their own decomposition which results in a net gain of
nutrients available to the seagrasses, and the bacteria and fungus
themselves are food for many other animals in the form of detritus.
The nutrient net gain is further enhanced by the geochemistry
that occurs within the sediment as briefly discussed above
concerning nutrient availability per sediment composition and grain
size.
The microbial mats found on the surfaces of both the
sediment and the leaves of the seagrasses are composed primarily of
cyanobacteria that have a dual role related to productivity by fixing
carbon dioxide and atmospheric nitrogen which often limits primary
production in many other ecosystems (Hamisi 2004). The
cyanobacteria found in such mats also provide food to the heterotrophs.
The inorganic nitrogen released by the heterotrophs utilizing the
cyanobacteria, supports continued primary production by seagrasses in
another cycle.
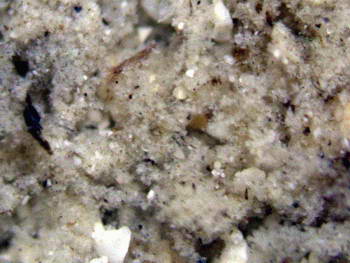
As the seagrass leaves are decomposed they release both particulate
and dissolved carbon and organic matter, which the bacteria and fungus
assimilate and transform into detritus (also known as marine snow), a
nutritionally important food source for detritivores. With
a wide range of animals that consume detritus in all habitats
throughout the oceans, it is of no surprise that given the massive
production found within seagrass meadows the diversity of detritivores
is equally as massive.
Examples of some common detritivores both below and above the sediment.
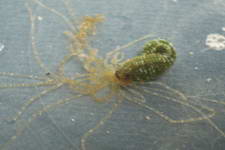
Nematode sp.
Cirratulid sp.
Polychaete sp.
Terebellidae sp.

Cephalochordata sp.
Foraminiferan sp.
Synaptid sp.
Holothuridea sp.
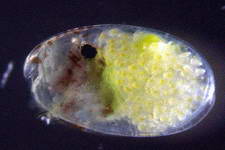
Copepod sp.
Amphipod
sp.
Isopod sp.
Ostracode sp.
As each animal consumes and
then digests the detritus, a fraction of the digested food, mostly
the amino acids and protein fragments will be used by the
organism to build or repair tissues. Some of this will eventually
be recycled and eliminated from the organism's body as ammonium in
urine. The rest of the digested foods, primarily the
carbohydrates and most of the lipids will be utilized in
cellular respiration, oxidized to produce energy. Eventually they
get eliminated from the organism as carbon dioxide and water (
Shimek,
2002).
The Grazers - Of seagrasses and epiphytic algae
In
a previous study (
Thayer 1984)
done on the effects of large herbivores
feeding upon seagrass productivity, the large herbivores were found
capable of exerting an influence on the seagrass nutrient web and the
stimulation of seagrass growth. Fish, sea turtles, sea
urchins and dugongs that graze directly upon the
seagrasses, representing at least 10% of their diet, can
significantly alter the
nutrient and detrital pathways by exporting the nutrients out of the
seagrass meadows by swimming away and defecating elsewhere. Their
grazing can also have both a stimulatory and negative impact on plant
production affecting community structure and function.
I have
observed that the once much more abundant local large herbivores no
longer
have a significant effect on the seagrass community, the numbers of
such grazers have been greatly reduced or eliminated by human
activities within the relatively shallow areas that lack any
enforcement of management regulations. With the uncontrolled
harvesting, the local seagrass nutrient web has lost an important
nutrient export link through the elimination of their primary
herbivores. This is a pandemic problem.
In areas where grazing by large herbivores still occurs,
the seagrasses have a below ground reserve of available nutrients which
allows the seagrasses to recover rapidly to levels that equal or exceed
those in nearby ungrazed beds. In areas of intense grazing, these
reserves have a stabilizing influence by allowing the seagrass to
persist as their rhizomes and roots are largely left intact and able to
quickly produce more leaves (
Valentine 1999). Such grazing
contributes much more to the transportation and disturbance of seagrass
nutrients elsewhere than is found to occur locally in this study area.
The local removal of herbivores and detritivores With
the loss or significant reduction of all local large herbivores due to human predation, the only
remaining major herbivore with any significant population is the
inedible (to humans)
Diadema sea urchin. During my
translocation study
of this species I was able to determine that adult sea
urchins restricted themselves to the immediate area surrounding their
shelter, only venturing out during darkness to graze within a meter or
two of their daytime shelter. Such self-restriction limits their
impact on seagrass to only those sea urchins that have found suitable
shelter on the edges of the seagrass meadows or in the deeper
depressions within the seagrass meadows that contain a suitable
rocky substrate in which to gain shelter from. Those depressions that
do contain sea urchins graze most macroalgae from the hard
substrate as well as the seagrasses that extend into the depression.
This constant clearing of all algae and plant growth creates suitable
conditions for the settlement and growth of a number of coral species,
that in their growth provide more substantial shelter for the sea
urchins. Is this the birth of a shallow inshore reef?
During the first two months after the end of the
monsoon season, large numbers of
Diadema setosum gather together and
roam across the seagrass meadows grazing upon the epiphytic macroalgae
species clearing their path of such growth while releasing some of the
macroalgae nutrients back into the seagrass ecosystem as waste and detritus.
Such congregations are what I believe to be the sea urchin's strategy
to ensure a mate is always nearby while also having an abundant and
readily available food source to gain or regain the energy and
nutrients expended by sperm/egg production.
The type of shelter required by Diadema setosum and their impact on nearby seagrasses
Astralium okamotoi
is the most abundant of the gastropods within the local seagrass
meadows, not selective in its feeding, leaving only the encrusting species behind.
Other commonly found snails include the
Euplica sp.,
Trochoidea sp. and the
Cerithidae sp.
Phanerophthalmus smaragdinus is
one of many herbivorous slugs, possibly a detritivore as I only find
them amongst the leaf litter where they can avoid predation.
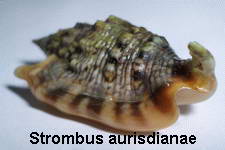
The only large gastropod found, feeding upon the epiphytic and
drift macroalgae that it can reach as it is restricted to the floor of
the meadow due to its size. Its movement on and in the leaf, detritus
litter and sediment helps to distribute nutrients through disturbance.
Human collection for food has greatly reduced their numbers.
Salarias fasciatus
also known as the lawnmower blenny is the most numerous of the
herbivorous fish with small juveniles found amongst the leaf litter
making forays up to the seagrass blades to forage the epiphyte algae
growth. During periods of high tide, schools of both adult and
juvenile rabbitfish species enter the seagrass meadows to graze upon
drift Ulva spp. and seagrass epiphyte growth.
The herbivores shown above are only a sample of the most commonly found
species, there are of course far to many others for me to include.
The Larger Predators - Some are transitory, others are full time residents.
Both
fish and invertebrate species find the seagrass meadows to be rich
hunting grounds. Many fish species, especially larger predators, are
transient residents as the seagrass beds become too shallow for them
during low tides.
Invertebrate predators such as this
Archaster sp.
(sand sifting starfish) are permanent residents of the seagrass beds as
they consume the infauna of the sediment. Other large
invertebrate predators include most other starfish species, hermit
crabs, the swimming crabs and many other crustaceans.
Fish Predators such
as this pipefish are also abundant given the high productivity of
the seagrass ecosystem. As shown above, fish such as this
pipefish species are clearly full time residents, evident by their
coloration and markings allowing them to blend in with the seagrass.
File fish species also take the same colorations and markings while
the flamboyantly colored fish species make themselves obvious as to
their having come into the seagrass meadows from the coral reefs and
are thus transitory opportunists.
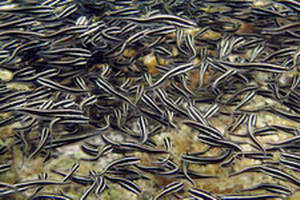
Schools of both juvenile and subadult
Plotosus lineatus (striped sea catfish) are a common sight as they leap frog over each other sifting detritus and sediment infauna.
Local Seagrass Distribution
-
The various hues of green in the below photograph are not entirely due
to seagrass growth. The healthy seagrass meadows are found between
the shoreline out to the 2 meter depth range, beyond that depth the
frondose macroalgae dominate with an outer band of kelp growth prior to
the coral reef. The seagrasses found at depth prior to the
coral reefs are at their toleration limits having shorter and fewer
leaves with individual plants widely spaced in comparison to those
plants found in the shallows.
As shown below the vast flat expanse greatly reduces the wind driven
waves and slows the effect of tidal flows providing a shallow,
sheltered and near calm environment critical to the formation of
composting leaf and detritus mats that are responsible in large part
for the high productivity of seagrass meadows.
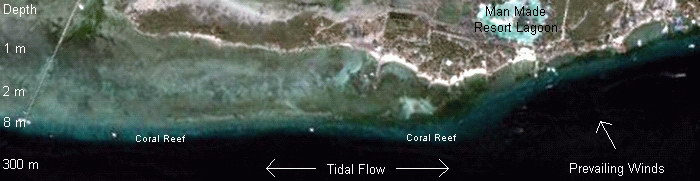
Seagrass distribution of Mactan Island, The Philippines
Disturbances - Weather, water movement and fauna
As
mentioned throughout this article, the feeding activities and movements
through the seagrass, detritus and sediment as well as climatic and
tidal events cause disturbances. These disturbances of the
nutrients are yet another important factor in the seagrass nutrient
web. During periods of storm activity the
larger than normal wind driven waves can uplift and suspend the leaf
and detritus litter, moving large amounts of organic matter either
towards shore or far out to sea with the tides and making
the nutrients available to a large number of other animals outside of
the seagrass habitat. After periods of unusually strong winds and
high waves, the shoreline can accumulate large mounds of wind and wave
driven leaf litter that decomposes on shore releasing nutrients
that wash back into the ocean through rainfall runoff spurring the
growth of shoreline filamentous algae and thereby transporting the
seagrass productivity elsewhere.
Such transportation also occurs when the prevailing tide and winds
carry the leaf litter and detritus out to sea and deposits it onto the
coral reef. Carried far enough, the organic material can find its
way to the deep ocean and drift downwards thousands of feet, being
consumed and broken down further by pelagic plankton and fish and
microbial action in the deep benthos, only to be carried back to the
surface again in areas of ocean upwelling. Upwellings then fuel the
production of plankton, contributing once again to the recycling of
nutrients made available to the coral reef inhabitants.
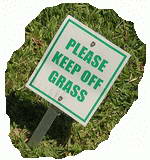
Complex.
The only single word that best describes the diversity and
nutrient webs that the seagrass meadows provide. Doing the
research for this article has made me much more aware of what used to
be a little thought of habitat, giving me a greater appreciation
and a sense of gratitude that the seagrass meadows are where they are.
Without such meadows, the coral reefs that we tend to focus on
would be less for it.
Come Study and Learn of this and other habitats !


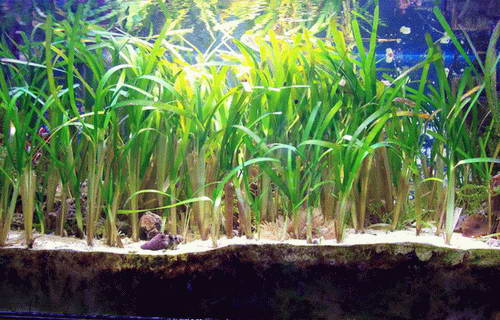
Clay & Mollie Dible's beautiful Seagrass Refugium
Having explored a natural seagrass meadow we now turn our attention to
the keeping of seagrass within an aquarium. Given the conditions and
the nutrient dynamics discussed pertaining to natural meadows we now
have a greater understanding of their needs and what they can bring to
a reef aquarium system. When used as a refugium plumbed into a
reef display aquarium the nutrient processing and production of
an aquarium reef system is enhanced and negates the need to use
equipment and other products that attempt to do what nature does best, if allowed to.
Sediments
- The seagrass refugium's sediment is vital to the health and
long term survival of the seagrass just as it is in natural meadows.
How you construct the sandbed is going to determine its
functionality in providing a nutrient rich environment for the
seagrasses rhizomes and root structures. Seagrasses
are plants that depend upon their roots for the uptake of
nutrients, roots that require extremely fine grain sizes, it
will be imperative that a calcium carbonate substrate with grain sizes
ranging from 0.2 - 1.02mm be used with a depth of no less than six
inches, deeper if possible. Incorporating a live mud into the
sediment during the sand beds construction will ensure a
suitable number of infauna are introduced. Just as in nature the
infauna are critical to the functionality of any sandbed to process
nutrients and prevent the formation of sand clumps by their movement
through the sediment (Shimek 2001).
Patience -
With the refugium plumbed inline with the rest of the aquarium
system now comes the most difficult part of it all. The wait. It
will take at least six months to one year for the sediments to have
become enriched enough to support the requirements of the seagrasses
(Calfo 2005). To rush the introduction of the plants will most
likely ensure their loss. Having been uprooted, transported and
their root systems likely damaged, the plants will need everything to
be in their favor to get off to a good start.
Indo-Pacific Plant Selection
- With the construction of the sandbed having been completed you
now have at least six months to determine which species are available
to you and study their husbandry. My experience with any of the
seagrasses is limited to the indo-pacific species yet through my study
and recent attempts at keeping indo-pacific seagrasses it appears they all share
some basic needs and care requirements, evident by their all being
found within the same seagrass meadow.
Halophila ovalis
appear to be the most tolerant of less than ideal handling
and capable of surviving being shipped with bare roots and wrapped
in moist paper towels for a number of days (Borneman 2008). Being
a pioneer species may account for this hardiness as they
are frequently the first species to grow into uncolonized
soft substrates. This apparent ability to go where no plant has gone
before
would in my opinion make them the best candidate for establishing a
seagrass habitat with the later introduction of other seagrass
species. Their very short growth and relatively low lighting needs
in comparison to other seagrasses make them ideal for placement in
coral reef aquariums as
there is no danger of this seagrass shading or becoming abrasive to the
corals and will tolerate the lowered light intensity found at the
aquarium's sediment level.
Thalassia hemprichii - While not as common as
T. testudinum (shown in the photo),
the
two species share very similar morphology and husbandry requirements.
Given such similar morphology, I doubt many hobbyists can distinguish
between the two other than by knowing where they were collected. With
T. testudinum
being an Atlantic species it is most likely that those hobbyists in the
United States will use this species as their first seagrass keeping
attempt(s) as it would be the most readily available of
the species. While not impossible to maintain, this species
does appear to be sensitive to uprooting and the
subsequent exposure to air.
Syringodium isoetifolium - Second only to the
Halophila sp. in its ability to colonize. I have found
S. isoetifolium to be hardy and fast growing. It transplants much easier than the
Thalassia sp.
with a high rate of survival. This species would make a good
addition to either a newly established or mature seagrass aquarium,
able to colonize rapidly while making a suitable companion species with
established
Thalassia spp.
Normally not growing as tall as the Thalassia, it is not affected by
partial shading and with their very thin, tubular leaves they pose no
risk of shading the wider bladed
Thalassia spp. either.
These traits between the two genera may explain their combination
being the dominant structures in natural seagrass meadows here in the
Philippines.
Enhalus acoroides - With
leaves averaging a length of 130cm and 3cm wide with root systems that
can extend well beyond 30cm deep, this species is not a realistic
choice for home aquarium systems. For scale, the floor tiles
shown in the photo are each a square foot with the plant being so long
that I had to stand on a chair to get the entire plant into the frame.
However, if one were to set up a suitable aquarium for this species it
would make for a very unique display. The affect of such a
planted aquarium would be reminiscent of a kelp forest.
Lighting the Seagrass Aquarium
- Given the environment the seagrasses are found in as discussed
previously it should be obvious that they require high light
intensity to thrive. Estimates from various studies (Kenworthy 1996)
done on the minimum lighting requirements of seagrass species have
shown that their lower toleration limit to be in a range from 24% to
37% of the light just beneath the water surface which equates to
photosynthetically active radiation (PAR) levels of between 200 to
600 PAR at wavelengths between 400nm and 700nm. Levels below these
minimums result in the seagrasses producing shorter and fewer leaves,
obvious indicators that more light intensity is needed over the
aquarium. Extended periods of light levels below their minimum
requirement places a stress on the seagrasses that some species are
unable to recover from.
Halophila spp. are the exception and have much lower lighting requirements.
Since most hobbyists have their own preferences in the lighting systems
that they use, I am not going to suggest any particular type of
lighting and instead stress again the importance of light
intensity to the seagrasses. How that intensity is provided is
irrelevant unless it has an impact on animals being kept within the same system due to heating of the water.
Water Flow & Temperature - Tropical
seagrasses have a high thermal tolerance averaging 90 degrees fahrenheit and live close to
their thermal limits in the shallow, protected environments they
are found in. An aquarium that maintains the average
Indo-Pacific coral reef water temperature of 82F is well within the
temperature range that tropical seagrasses are adapted for, those
aquariums that maintain lower than normal coral reef
temperatures may encounter slower seagrass growth and their
failure to thrive. Temperature is second only to light intensity
pertaining to tropical seagrasses primary needs.
Water
flow through the seagrass aquarium should not include the use of
powerheads or other high flow pumps and instead simply allow the
overflow volume from the rest of the system to provide bulk water
movement that will not suspend the leaf litter and detritus and
encourage diversity of fauna and infauna.
Water Parameters
- Being a plant, the seagrasses utilize the same common nutrients
as terrestrial plants. Nitrogen, phosphorus, iron and carbon
dioxide, most of which is absorbed by the roots from the sediment with
the exception of carbon. Phosphorus will not normally be a limiting
factor in an aquarium system due to the input the system receives
through the addition of food. Nitrogen and iron is more likely to
be utilized first by algae and bacteria and may become limiting to the seagrasses.
These limitations can be overcome by the use of plant food sticks
or tablets (Borneman 2008) pushed down into the sediment near the
plant's roots. It is unlikely that nutrients provided in this
manner will have any affect on the system unless the sediment is
disturbed allowing the release of the nutrients into the circulating
water.
Planting the Aquarium
- Now that the long wait is over with the sediment having been
given time to sequester nutrients and the plant species selected for
planting, its time to break out the gardening tools and... actually
you will need nothing more than your gloved hand to accomplish the
delicate task of placing your seagrasses into the sediment.
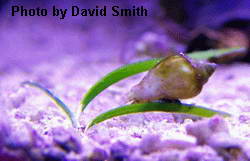
The roots of seagrasses are fragile with any
damage done being the biggest factor in losing purchased
plants. This
is unavoidable when purchasing seagrasses from commercial sources as
the roots are usually stripped of any sediment to lessen the shipping
costs involved with heavy sediments (Calfo 2005). A good reason
to start out with the hardier species that are
known to have a relatively high survival rate when transported in such
a manner. If seagrasses are being shared or purchased from a
local established seagrass aquarium then you have the opportunity to
collect individual plants with less damage or disturbance to the roots
by gently moving the sediments to expose the rhizome and cutting the
rhizome with scissors in six inch lengths. Once cut, gently lift
the plant so as to keep as much of the root attached sediments intact
and place the plant in a suitable container while being held under
water (Borneman 2008).
Having collected seagrasses from the
local meadows, I have found that it is much easier to properly plant
such lengths of the seaweed by simply making a trench in the aquarium's
sandbed and gently place the rhizome and its roots into the trench and
cover with sediment. Do not force or push the rhizomes into the
sediment as it will only break the rhizome and cause further damage.
Again, having access to the local seagrass meadows means that I
can take extra measures to tip the plants survival rate in my favor.
A shallow, wide tupperware container and a spatula allows me to
lift entire sod sections containing the rhizomes, roots and all of
its surrounding sediment gently into a tupperware container (all done
underwater) for easy transport back to my aquarium. Digging a
suitably sized pit into the sandbed I can then lower the tupperware
onto the sediment and gently slide the entire sod section into the pit
and cover with a centimeter or two of sand.
Once planted,
it is not uncommon for the seagrasses to drop all of their leaves due
to the shock of having been disturbed. With their relatively fast
rate of leaf production, new leaves should begin to emerge within a
week or two at most. You can help ensure the plant has
additional nutrients to replace its lost leaves and to recover
more quickly by following a tip from Eric Borneman who has had
increased success with newly planted seagrasses by purchasing
freshwater plant food tablets that are broken in half and pushed down
into the sediment close to the plant's root structures.
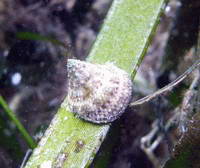
As with any available lighted surface, microalgae will grow upon
the seagrasses leaves and shorten the leaves usefulness to the plant
by blocking the available light. With
Astralium spp.
being the most commonly found snail consuming the microalgae on
seagrass blades in natural meadows and being the most commonly sold
species, they would make the best choice for keeping your seagrasses
clean of epiphytic microalgae. A good stocking number to start out with
would be one snail per plant, increasing their numbers if you find that
the snails are unable to keep up with microalgae growth. I feel I
should point out that these species are most often sold to the reef
aquarium hobby not because they are suitable for our rocky coral
displays, they are not, but simply because they are found in great
numbers in the seagrass meadows and with the meadows being nearshore
and easily accessed they are collected by simply wading through the
meadow and picking them off the seagrasses without any need for scuba
gear as is required to collect the snail species that are found in
coral reef areas.
Conclusion :
With what I have learned and observed of a tropical seagrass
meadow it became obvious that a suitably sized refugium
containing a
live, deep sand bed constructed with calcium carbonate sediment of the
correct grain sizes and stocked with seagrasses in a specific species
sequence, will provide a diverse and functional habitat
allowing a reef aquarium system an enhanced capability.
Related Reading
:
A Philippine Fringing Reef & The
Reef Aquarium Part One A Philippine Fringing Reef & The Reef Aquarium Part Three A Philippine Fringing Reef & The Reef Aquarium Part Four
An Online Philippine Reef Tour
The Reef Aquarium Clean Up Crew Acknowledgments :
I would like to thank my wife Linda for her loving
support and understanding of my interests in all things marine. A
special thank you goes out to Eric Borneman for his generosity in
providing assistance with this article and in helping me to make sense
of tropical reefs. To Dr. Ron Shimek and Leslie Harris, thank you for
the many identifications made as well as teaching me a great deal about
marine biology and zoology.
References:
Bell S.S. et al. (1997), Drift Macroalgal Abundance in Seagrass beds, Mar Ecol Prog Series, Vol. 147:277-283
Borneman E.H. (2008), Sensational Seagrasses, Marine Fish and Reef publication, Vol. 10
Calfo
A. (2005), Beautiful Seagrasses - Keeping True Flowering Plants in Your
Marine Aquarium, http://www.reefland.com/rho/0305/main3.php
Capone
D.G. et al. (1992) Microbial nitrogen transformations in unconsolidated
coral reef sediments, Mar Ecol Prog Series, Vol. 80: 75-88.
Eckman,
J. E., Nowell, A. R. M. and Jumars, P. A. 1981. Sediment
destabilization by animal tubes. Journal of Marine Research. 39:
361-374.
Erftemeijer P.L. (1993),
Sediment-Nutrient interactions in tropical seagrass beds. Mar Ecol Prog
Series, Vol. 102: 187-198.
Fitzpatrick
J. et al. (1995), Effects of prolonged shading stress on growth and
survival of seagrass Posidonia australis, Mar Ecol Prog Series, Vol.
127: 279-289
Hamisi,
M.I. et al. (2004), Cyanobacterial occurrence and diversity in seagrass
meadows. Western Indian Ocean J. Mar. Sci. Vol. 3, No. 2, pp.
113–122, 2004
Hansen
O.G. et al. (1992), Growth rates and photon yield of growth in natural
populations of a marine macroalga Ulva lactuca. Mar Ecol Prog Series,
Vol.81: 179-183
Kenworthy,
W.J. et al. (1996), Light Requirements of Seagrasses Halo&de
wrightii and Syringodium filiforme Derived From the Relationship
Between Diffuse Light Attenuation and Maximum Depth Distribution.
Estuaries Vol. 19, No. 3, p. 740-750
Lardizabal S.
(2006), Beyond the Refugium: Seagrass
Aquaria, http://reefkeeping.com/issues/2006-04/sl/index.php
McGlathery,
K. J., Howarth, R. W., Marino, R. (1992). Nutrient Limitation of the
macroalga, Penicillus capitatus, associated with subtropical seagrass
meadows in Bermuda. Estuaries 15: 18-25
Moriarty,
D.J. et al. (1990), Primary and bacterial productivity of tropical
seagrass communities. Mar Ecol Prog Series, Vol. 61: 145-157
Shimek
R.L. (2003), How Sandbeds Really Work,
http://www.reefkeeping.com/issues/2003-06/rs/feature/index.php
Shimek
R.L. (2001), The Importance of Deep Sand,
http://www.ronshimek.com/Deep%20Sand%20Beds.htm
Shimek R.L. (2002), The Infamous Detritivores, http://www.reefkeeping.com/issues/2002-03/rs/index.php
Short
F. T. (1987). Effects of sediment nutrients on seagrasses: literature
review and mesocosm experiment Aquat. Bot. 27: 41-57
Stapel
J. et al. (1996), Nutrient uptake by leaves and roots of the seagrass
Thalassia hemprichii, Mar Ecol Prog Series, Vol. 134: 195-206
Sullivan
M. 1994. The taxonomy of seagrasses surveyed from higher taxa down
through the family level. Florida Int. Univ.
http://www.fiu.edu/~seagrass/class/bot5647/maureen.htm.
Tanaka
Y. et al. (2007) Interspecific variation in photosynthesis and
respiration balance of three seagrasses in relation to light
availability. Mar Ecol Prog Series, Vol. 350: 63–70.Thayer G.W. et al. (1984), Role of Larger Herbivores in Seagrass Communities, Estuaries vol.7, #4, 351-376.
Thomas
F.M. (2003), Ammonium uptake by seagrass communities: effects of
oscillatory versus unidirectional flow, Mar Ecol Prog Series, Vol. 247:
51–57
Valentine,
J.F. et al. (1999), Seagrass Herbivory: Evidence for the Continued
Grazing of Marine Grass. Mar Ecol Prog Series, Vol.176: 291-302
Vermaat
J.E. et al. (1995) Meadow maintenace, growth and productivity of a
mixed Philippine seagrass bed. Mar Ecol Prog Series, Vol.124: 215-225.
© 2011 All Rights Reserved
All content and photographs are copyright protected and may not be
used or reproduced in any manner without written permission of the authors.